The existence of viruses in the history of science is robustly supported by a vast and always growing body of scientific research, underscoring their significance in biology and medicine. This article avoids contemporary examples due to controversy and focuses on established science history. It will not evaluate if current systems were abused to mislead the public, but it will examine if viruses exist. We have read many claims that they do not and we have considered them from an open minded fact-based perspective. For example, what about vacuoles? See below for that. But first, let’s go over the best evidence. Here are some compelling pieces of evidence that affirm the presence of viruses.
Evidence for the Existence of Viruses
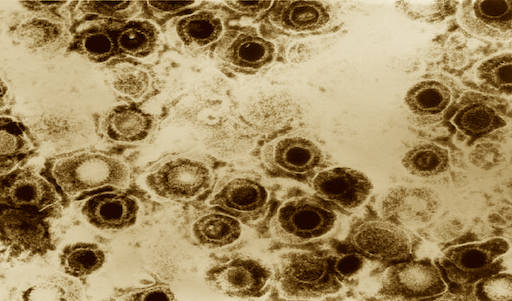
1. Direct Observation
Experiment: The tobacco mosaic virus (TMV) was one of the first viruses ever studied in detail. In 1935, a scientist named Wendell M. Stanley managed to crystallize TMV, which means he turned it into a solid form that could be analyzed. By using special X-ray techniques, Stanley discovered that TMV has a simple structure made up of RNA (a type of genetic material) and protein. This breakthrough was important because it provided clear evidence that viruses are actual particles, not just liquids or something unclear. Stanley’s work helped lay the foundation for the field of virology, which is the study of viruses. His discovery showed scientists that viruses could be studied in a more organized way, leading to a better understanding of how they work and how they can affect living things.
2. Infectious Nature
Experiment: The infectious nature of viruses was clearly shown through experiments with the tobacco mosaic virus (TMV). In the early 1900s, scientist Martinus Beijerinck conducted important experiments where he filtered infected sap from tobacco plants to isolate the agent causing the disease. He discovered that even after filtering out all visible microorganisms, the sap could still infect healthy plants. This proved that the cause of the disease was not a bacterium, which is larger and would have been caught in the filter, but a smaller entity that was later identified as a virus.
Beijerinck’s work demonstrated that this mysterious agent could reproduce itself in living plants. He repeated his experiments multiple times and found that the disease-causing power of the sap remained strong, even after being diluted. This was significant because it showed that the infectious agent was alive and could multiply, unlike a chemical that would lose its strength when diluted. Beijerinck concluded that this new type of infectious agent was something entirely different from bacteria, which he called a “virus.” His research laid the groundwork for the field of virology, helping scientists understand viruses better and paving the way for future discoveries.
3. Genome Sequencing
Experiment: Understanding the structure and function of viruses has greatly benefited from sequencing their genomes. For example, in the late 1970s and early 1980s, scientists sequenced the genome of bacteriophage φX174, which was one of the first DNA viruses to be studied in this way. This work revealed important details about how viruses replicate and how their genetic material is organized. By sequencing φX174, researchers provided direct evidence that viruses have their own genetic material, which is crucial for understanding how they operate and interact with living cells. This groundbreaking research helped pave the way for future studies in virology, allowing scientists to learn more about how viruses work and how they can affect health.
4. Viral Replication
Experiment: The replication cycle of viruses has been studied in detail using different models, and one of the key examples is the poliovirus. Researchers have conducted experiments to understand how poliovirus attaches to and enters host cells. They used special techniques, such as labeling the viral RNA with radioactive markers, to track its journey into healthy cells and how it replicates once inside.
These experiments showed that poliovirus relies on the machinery of the host cell to reproduce itself. When the virus attaches to a specific receptor on the surface of a host cell, it undergoes changes that allow it to enter the cell. Once inside, the viral RNA is released and begins to use the cell’s resources to make more copies of itself. This research confirmed that viruses cannot replicate on their own; they need a living host cell to do so. Understanding this process is crucial for developing treatments and vaccines against viral infections.
5. Virus Particles
Experiment: Scientists have developed various purification techniques to isolate viral particles from infected tissues. One classic method is ultracentrifugation, which uses very high speeds to separate viral particles from cellular debris in infected plants or animals. By spinning the samples at extremely high forces, researchers can effectively isolate viruses from other materials. In addition to ultracentrifugation, scientists also use techniques like enzyme-linked immunosorbent assays (ELISA) to detect specific viral proteins. ELISA works by using antibodies that specifically bind to viral proteins, confirming the presence of viruses in the samples. This combination of methods allows researchers to accurately identify and study viruses, providing important information for understanding viral infections and developing treatments.
6. Vaccination
Experiment: The development of vaccines against various viral diseases provides strong evidence for their existence. For example, Edward Jenner’s work in 1796 with cowpox led to the smallpox vaccine, demonstrating that exposure to a related virus could confer immunity against smallpox. Subsequent vaccines for diseases like measles and polio have shown consistent efficacy in preventing infections, further confirming the existence and impact of these viruses.
7. Antiviral Medications
Experiment: The development of antiviral medications has been based on understanding specific viral components. For instance, studies on the antiviral drug acyclovir demonstrated its effectiveness against herpes simplex virus (HSV). Researchers showed that acyclovir selectively inhibits viral DNA synthesis by targeting the viral enzyme thymidine kinase, highlighting how antiviral drugs can specifically interact with viral mechanisms.
8. Evolutionary Evidence
Experiment: Evolutionary studies have provided insights into the ancient origins of viruses through paleovirology. Researchers analyze integrated viral sequences found in the genomes of various organisms—these sequences are remnants from past infections that can indicate historical interactions between hosts and viruses. For example, studies have identified endogenous retroviruses (ERVs) within mammalian genomes that provide evidence for ancient viral infections and their evolutionary impact on host species over millions of years.
9. Money Spent on Research
Let’s say you choose not to believe anything coming from the universities. This is an absurd thing to do, but even if you did that, there is evidence from the military. From the inception of the U.S. biological weapons program in 1943 until its termination in 1969, the United States invested approximately $400 million in research and development for biological weapons. This significant financial commitment underscores the seriousness with which the military approached the potential threats posed by biological agents. It is unlikely that the U.S. military would allocate such extensive resources over nearly three decades to study something that was non-existent. The competition for military funding relies heavily on demonstrating tangible results, indicating that this was not a mere façade or scam. The sustained investment and the establishment of multiple laboratories indicate a genuine commitment to understanding and developing capabilities related to biological agents, including various viruses, which were recognized during that era as real threats.
But what about..
Are Viruses Just Vacuoles?
Vacuoles are structures found in the cells of plants and some other organisms. They act like storage containers, holding water, nutrients, and waste products. When looking at cells under a microscope, it’s important to be able to tell vacuoles apart from viruses, especially since some people mistakenly think that viruses are just vacuoles or similar structures.
To distinguish between vacuoles and viruses under a microscope, scientists often use electron microscopy. This powerful technique provides very detailed images of tiny structures that regular light microscopes cannot capture. Here’s how you can tell them apart:
1. Size: Vacuoles are generally larger than viruses. While vacuoles can vary in size, they are typically several hundred nanometers to micrometers across. In contrast, viruses are much smaller, usually ranging from about 20 to 300 nanometers.
2. Structure: Under an electron microscope, vacuoles appear as large, clear spaces filled with fluid or semi-solid material. They have a membrane surrounding them. Viruses, on the other hand, appear as small particles with distinct shapes (like spheres or rods) and are often surrounded by a protein coat called a capsid.
3. Content: Vacuoles contain various substances like water, sugars, and waste materials. In contrast, when examining infected cells, you might see viral particles inside vacuoles or near them, but these particles will look different from the vacuole itself.
4. Staining Techniques: Scientists can use specific staining methods to highlight viral proteins or structures within cells. For example, negative staining can help visualize viruses more clearly by making them stand out against the background.
By using these techniques and observations under an electron microscope, researchers can accurately identify whether they are looking at vacuoles or viral particles in a sample. Understanding these differences is crucial for studying cell biology and virology effectively.
Are Viruses Just Cellular Debris?
But what about the argument that viruses are just harmless byproducts of cellular processes? Some people claim that what we identify as viruses are actually just cellular debris or proteins that our bodies create and that they don’t cause disease. They argue that if viruses were truly harmful, we would have seen more consistent and severe effects across all individuals infected with them.
Under an electron microscope, it is clear that viruses and cellular debris are distinct entities. For example, studies have shown that viral particles, such as those from bacteriophages, exhibit specific shapes and sizes that are easily identifiable. The imaging techniques used, like scanning electron microscopy (SEM) and transmission electron microscopy (TEM), allow researchers to visualize the unique structures of viruses, including their capsids and surface features. In contrast, cellular debris appears as irregular fragments without the defined structures seen in viruses.
Research has shown that viruses have specific structures and genetic material that trigger immune responses. For example, experiments with the poliovirus demonstrated that it actively hijacks host cell machinery to replicate, leading to observable disease symptoms. In contrast, cellular debris does not elicit the same immune response; it is typically cleared away by the immune system without causing harm.
Furthermore, studies on viral virulence have shown that different viruses can vary widely in their ability to cause disease. For instance, research on bacteriophages has confirmed that virulent strains can outcompete less virulent ones in certain conditions, indicating a direct link between a virus’s characteristics and its impact on host organisms. Experimental work has also demonstrated that some viruses evolve higher virulence under specific conditions, which directly correlates with increased disease severity in infected hosts.
Are the Few People with Electron Microscopes Making Viruses Up?
While it’s difficult to provide an exact number of electron microscopes in use worldwide, a reasonable estimate would be in the range of 20,000 to 30,000 electron microscopes.
- Research Institutions and Universities: Thousands of universities and research institutions globally have dedicated electron microscopy facilities, each typically housing multiple electron microscopes. Are there even thousands of universities? Yes, as of 2024, there are more than 29,338 universities worldwide (unconfirmed), so if that is not an AI hallucination, then thousands of them having electron microscopes is possible.
- Industrial Applications: Many industries, particularly in fields like materials science, pharmaceuticals, and semiconductor manufacturing, also utilize electron microscopes for quality control and research purposes.
- Market Growth: With the increasing demand for advanced imaging techniques in various scientific fields, the number of electron microscopes is likely to grow steadily.
How many people have ever used an electron microscope to view a virus? Electron microscopy (EM) has been a key tool in virology since the 1940s. Thousands of researchers in virology, microbiology, and related fields have utilized EM to study viruses over the decades. A rough estimate could be that over 50,000 individuals have used electron microscopes specifically for viewing viruses throughout the history of this technology.
Is Virology A Scam? How Can We Know?
Viruses are real entities that exist in various forms, each exhibiting distinct and recognizable shapes. Over the years, numerous images of viruses have been captured using electron microscopes, providing visual evidence of their existence and diversity. One of the landmark studies in virology involved tobacco plants. Researchers took diseased tobacco leaves and filtered the extract to isolate particles smaller than bacteria. When this filtered juice was applied to healthy tobacco plants, those plants became infected and developed the same disease symptoms. However, when an even finer filter was used to remove all virus-sized particles from the liquid, the remaining solution did not cause any disease in the healthy plants. This experiment demonstrated that the infectious agent was something smaller than bacteria but larger than simple liquid components.
The Tobacco Mosaic Virus (TMV) was identified as the causative agent of this disease, leading to significant economic losses for tobacco growers. TMV is known to cause substantial economic losses in tobacco production, with estimates suggesting that it can lead to losses of approximately $2.5 billion annually worldwide . This financial impact motivated researchers to investigate and understand the nature of viruses, ultimately contributing to the foundational knowledge of virology.
Virologists developed effective strategies to prevent the spread of TMV in tobacco cultivation. These include:
- Sanitation Protocols: Implementing strict sanitation measures for tools and equipment to prevent cross-contamination.
- Employee Training: Educating workers on hygiene practices, such as frequent handwashing and prohibiting tobacco use in growing areas, to minimize the risk of introducing the virus into healthy plants.
Top Places to View Virus Images
Here are the top 10 places to view different virus images, based on the provided search results:
1. UTMB Virus Images
The University of Texas Medical Branch offers a collection of 22 electron micrographs of various viruses, including colorized versions. This resource is useful for educational purposes.
[View Images][42].
2. Foundations of Virology
This site provides access to a comprehensive eBook titled “Foundations of Virology,” which includes high-resolution images of viruses along with historical context and scientific discoveries.
[Explore the eBook] [43].
3. iStock Photo Library
iStock features a vast collection of nearly 2.2 million virus-related images, including stock photos of virus outbreaks and illustrations suitable for various projects.
[Browse iStock] [44].
4. Defense.gov High-Resolution Virus Pictures
The Department of Defense provides high-resolution images of viruses that are used in research and vaccine development, showcasing the visual aspects of various viral pathogens.
[Access the Collection] [45].
5. CDC Public Health Image Library
The Centers for Disease Control and Prevention (CDC) maintains a public health image library that includes a variety of virus images, particularly those relevant to infectious diseases.
6. National Institutes of Health (NIH) Image Gallery
NIH offers an extensive gallery featuring images related to virology research, including electron micrographs and illustrations of viral structures.
7. Nature Publishing Group
Nature publishes articles and studies that often include high-quality images of viruses as part of their scientific publications, available through their journal archives.
8. Virology Journal
This open-access journal frequently publishes research articles that include detailed images of viruses, providing both visual and contextual information about various viral studies.
9. European Molecular Biology Laboratory (EMBL)
EMBL provides resources and images related to molecular biology, including virus structures captured through advanced imaging techniques.
10. Science Photo Library
This commercial image library specializes in scientific imagery, including a wide range of virus photos suitable for educational and professional use.
These resources collectively provide a diverse array of virus images for educational, research, and professional purposes.
Conclusion
The cumulative evidence from direct observation, infectious capabilities, genomic analysis, replication studies, particle detection, vaccination success, antiviral efficacy, and evolutionary insights collectively supports the existence of viruses as critical biological entities that influence health and disease across diverse ecosystems.
Read More
[1] https://en.wikipedia.org/wiki/United_States_biological_weapons_program
[2] https://en.wikipedia.org/wiki/United_States_biological_defense_program
[3] https://www.ncbi.nlm.nih.gov/books/NBK209341/
[4] https://www.jstor.org/stable/24979407
[5] https://www.nsf.gov/awardsearch/showAward?AWD_ID=0218007
[6] https://www.clinicalmicrobiologyandinfection.com/article/S1198-743X(14)62634-3/fulltext
[7] https://www.jstor.org/stable/3092154
[8] https://bengaluru.sciencegallery.com/contagion-exhibits/wendell-stanley
[9] https://en.wikipedia.org/wiki/index.html?curid=357756
[10] https://en.wikipedia.org/wiki/Tobacco_mosaic_virus
[11] https://journals.plos.org/ploscompbiol/article?id=10.1371%2Fjournal.pcbi.1010423
[12] https://microbewiki.kenyon.edu/index.php/Tobacco_Mosaic_Virus_Uses_In_Pharmaceutical_Research
[13] https://nobelprizemuseum.se/en/the-first-virus-ever-discovered/
[14] https://pdb101.rcsb.org/motm/109
[15] https://plantpath.ifas.ufl.edu/classes/PLP4222-PLP6223/LECTURE%201%20INTRODUCTION/100YearsofVirology.pdf
[16] https://pmc.ncbi.nlm.nih.gov/articles/PMC1500891/
[17] https://pmc.ncbi.nlm.nih.gov/articles/PMC1563862/
[18] https://pmc.ncbi.nlm.nih.gov/articles/PMC4311501/
[19] https://pmc.ncbi.nlm.nih.gov/articles/PMC4442392/
[20] https://pubmed.ncbi.nlm.nih.gov/10470266/
[21] https://pubmed.ncbi.nlm.nih.gov/11570281/
[22] https://pubmed.ncbi.nlm.nih.gov/865612/
[23] https://www.aai.org/About/History/Notable-Members/Nobel-Laureates/WendellMStanley
[24] https://www.apsnet.org/edcenter/apsnetfeatures/Documents/1998/ZaitlinDiscoveryCausalAgentTobaccoMosaicVirus.pdf
[25] https://www.embopress.org/doi/10.1038/sj.emboj.7601831
[26] https://www.jstor.org/stable/56736
[27] https://www.nature.com/articles/267216a0
[28] https://www.nature.com/articles/nrmicro817
[29] https://www.nature.com/articles/s41598-021-87694-x
[30] https://www.rockefeller.edu/our-scientists/wendell-m-stanley/2538-nobel-prize/
[31] https://www.smithsonianmag.com/science-nature/what-are-viruses-history-tobacco-mosaic-disease-180974480/
[32] https://www.tudelft.nl/en/antoni-van-leeuwenhoekjaar/background/martinus-willem-beijerinck/the-professor/viruses
[33] https://www.frontiersin.org/journals/microbiology/articles/10.3389/fmicb.2020.02014/full
[34] https://www.frontiersin.org/journals/microbiology/articles/10.3389/fmicb.2018.03255/full
[35] https://www.frontiersin.org/journals/microbiology/articles/10.3389/fmicb.2020.00790/full
[36] https://inserm.hal.science/inserm-00308276/document
[37] https://mmegias.webs.uvigo.es/02-english/5-celulas/5-vacuolas.php
[38] https://www.youtube.com/watch?v=uM0yE8UXX0U
[39] https://pubmed.ncbi.nlm.nih.gov/38393367/
[40] https://pmc.ncbi.nlm.nih.gov/articles/PMC7169071/
[41] https://pmc.ncbi.nlm.nih.gov/articles/PMC2772359/
[42] https://www.utmb.edu/virusimages/the-virus-images
[43] https://www.utmb.edu/virusimages/
[44] https://www.istockphoto.com/de/bot-wall?returnUrl=%2Fde%2Fphotos%2Fvirus
[45] https://www.defense.gov/News/Feature-Stories/Story/Article/2115411/high-resolution-virus-pictures-help-researchers-develop-vaccines/