We know that CO2 in the atmosphere lets sun in, but traps the resulting heat that tries to leave the earth. This warms the planet. As CO2 and other greenhouse gases increase, so does the overall temperature. A warmer planet is not a problem, until the extra heat starts to change other things, eventually making your planet inhospitable to life.
That’s why we should all like new ways to turn CO2 into something useful, like fuel.
Carbon dioxide (CO₂) is essential for life but also a leading cause of global warming-the classic case of “the dose makes the poison.” Researchers at the University of Georgia have engineered a microbe, Pyrococcus furiosus, a heat-loving extremophile, to absorb CO₂ directly from the atmosphere and convert it into valuable chemicals and fuels. By genetically modifying the microbe to thrive at cooler temperatures and using hydrogen gas to drive a chemical reaction, the team enabled it to produce 3-hydroxypropionic acid, an industrial precursor for acrylics, and potentially other fuels. Unlike plants, which capture CO₂ through photosynthesis but require energy-intensive biomass processing, this microbe offers a more direct and efficient route to carbon-neutral fuels. When these biofuels are burned, they release only the CO₂ initially captured, making them a cleaner alternative to fossil fuels. While still in early stages, this breakthrough holds promise for scalable, cost-effective carbon capture and fuel production, potentially helping to mitigate climate change by turning atmospheric CO₂ into useful products.
About Pyrococcus furiosus
Pyrococcus furiosus is a hyperthermophilic archaeon known for thriving at extremely high temperatures, with an optimal growth temperature around 100 °C-hot enough to destroy most life forms. Classified as a hyperthermophile, it inhabits environments such as deep-sea hydrothermal vents. This microorganism is strictly anaerobic and heterotrophic, metabolizing carbohydrates through fermentation to produce CO₂ and hydrogen. Notably, P. furiosus possesses unique tungsten-containing enzymes, such as aldehyde ferredoxin oxidoreductase, which are rare in biology and function optimally above 90 °C. Its cells are roughly spherical, about 0.8 to 1.5 micrometers in diameter, and feature monopolar flagella made of glycoproteins that aid in motility, surface attachment, and cell-to-cell connections. P. furiosus’s unusual respiratory system generates energy by reducing protons to hydrogen gas, creating an electrochemical gradient for ATP synthesis-an ancient mechanism possibly ancestral to those in higher organisms. These distinctive properties make P. furiosus valuable for industrial and biotechnological applications, including thermostable enzymes used in DNA amplification and chemical production.
Is it a bacteria? No.
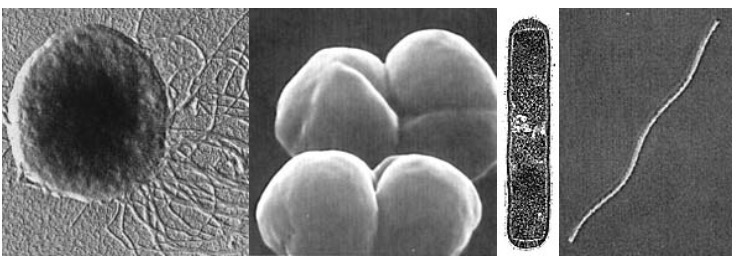
Archaea (pronounced R-K-uh) and bacteria are similar in size and shape, typically ranging from about 0.7 to 4 micrometers, and often appear as rods, spheres (cocci), or spirals. However, some archaea exhibit unusual forms, such as the flat, square-shaped cells of Haloquadratum walsbyi. Despite their morphological resemblance to bacteria, archaea differ significantly at the molecular level. Their genes and key metabolic pathways, especially those involved in transcription and translation, are more closely related to eukaryotes. Unique biochemical features include cell membranes composed of ether-linked lipids like archaeols, which provide stability in extreme environments. Archaea exploit a wider range of energy sources than eukaryotes, metabolizing organic compounds, ammonia, metal ions, or hydrogen gas. Some groups, like the salt-loving Haloarchaea, harness sunlight for energy, while others fix carbon, though no known archaeon performs both photosynthesis and carbon fixation like plants or cyanobacteria. Reproduction occurs asexually through binary fission, fragmentation, or budding, and unlike bacteria and eukaryotes, archaea do not form spores. These distinctive traits enable archaea to thrive in diverse and often extreme habitats, from hot springs to salty lakes.
Are Archaea Found in Humans?
Yes, archaea are present in and on the human body, but they are far less abundant than bacteria. Archaea inhabit various body sites including the skin, respiratory tract, gastrointestinal (GI) tract, and urogenital tract. For example, ammonia-oxidizing archaea (Thaumarchaeota) are common on the skin, while methane-producing archaea (methanogens) dominate in the gut and urogenital tract. Recent studies using advanced molecular techniques have found that archaea can make up roughly 0.5% to 5% of the microbial community in certain body sites, with some regions like the appendix and nose showing nearly equal archaeal and bacterial 16S rRNA gene counts. However, in most areas, bacteria vastly outnumber archaea; for instance, bacterial gene copies in the urogenital tract can be 26 to 3,900 times higher than archaeal gene copies. Overall, archaea represent a minor but consistent and diverse component of the human microbiome, with potential roles in health and disease that are still being explored.
Is Pyrococcus furiosus and Engineered Organism?
While it is not inherently engineered, modern genetic tools have enabled researchers to modify its genome for various biotechnological applications. P. furiosus is naturally competent, allowing the introduction of foreign DNA, and scientists have successfully created engineered strains that produce valuable chemicals such as 3-hydroxypropionic acid and 1-propanol by inserting synthetic operons into its genome. These engineered variants retain the microbe’s remarkable heat tolerance and metabolic versatility, making P. furiosus a promising platform for industrial processes operating at high temperatures. Thus, while wild-type P. furiosus is a natural organism, engineered strains have been developed through advanced genetic manipulation to enhance and expand its biochemical capabilities.
Pyrococcus furiosus was engineered to safely remove COâ‚‚ by introducing genes from the carbon-fixing archaeon Metallosphaera sedula that enable it to incorporate COâ‚‚ into valuable chemicals. Specifically, researchers inserted five genes from the 3-hydroxypropionate/4-hydroxybutyrate (3-HP/4-HB) carbon fixation cycle, allowing P. furiosus-which normally cannot fix COâ‚‚-to convert COâ‚‚ and acetyl-CoA into 3-hydroxypropionic acid (3-HP), a key industrial chemical. This engineered strain uses hydrogen gas as an electron donor to drive the reduction of COâ‚‚, effectively mimicking a biological carbon capture and conversion process.
The process operates at about 72°C, roughly 30°C below the microbe’s optimal growth temperature, which limits growth but maintains sufficient metabolic activity for CO₂ fixation. By using hydrogen as a clean energy source and coupling it with the introduced carbon fixation pathway, the engineered P. furiosus converts CO₂ into stable, useful products without producing harmful byproducts. This method bypasses the inefficiencies of photosynthesis by plants and biomass processing, offering a more direct and potentially scalable route to carbon-neutral fuel and chemical production.
In summary, P. furiosus was engineered by heterologous expression of carbon fixation genes to safely and efficiently convert atmospheric COâ‚‚ into industrially valuable compounds using hydrogen gas as a reducing agent, providing a promising platform for sustainable carbon capture and utilization.
Differences Between Archaea and Bacteria
Archaea and bacteria are both prokaryotes-unicellular organisms without a nucleus-but they differ significantly in their genetics, biochemistry, and cell structure. One key difference lies in their cell membranes: archaea have membranes made of ether-linked lipids containing isoprenoid chains (phytanyl), which can form lipid monolayers or bilayers, providing stability in extreme environments. In contrast, bacterial membranes are composed of ester-linked fatty acids arranged in lipid bilayers. Their cell walls also differ; bacteria typically have peptidoglycan, a polymer of sugars and amino acids, while archaea lack peptidoglycan and instead have cell walls made of pseudopeptidoglycan, polysaccharides, glycoproteins, or pure proteins.
Genetically, archaea share more similarities with eukaryotes than bacteria, particularly in DNA replication, transcription, and translation machinery. For example, archaea have multiple origins of replication and RNA polymerases more akin to those in eukaryotes. Archaea initiate protein synthesis with methionine, like eukaryotes, whereas bacteria use formyl-methionine. Additionally, archaea are generally resistant to antibiotics that target bacterial ribosomes.
Metabolically, archaea can utilize a wider range of energy sources, including organic compounds, ammonia, metal ions, and hydrogen gas. Some archaea (e.g., Haloarchaea) use sunlight for energy, but unlike plants or cyanobacteria, no archaea perform both photosynthesis and carbon fixation.
Structurally, archaea often resemble gram-positive bacteria with a single membrane and thick cell wall, but their unique biochemical features and evolutionary lineage set them apart as a distinct domain of life.
Bonus Strange Organism
Did you know there was a square microbe that grows in super salty pools all over the world? Haloquadratum walsbyi looks like a tech company logo.
Wow.
Anyway, here’s hoping the manmade version of the microbe Pyrococcus furiosus helps us recover from over carbonation. Carbon Nation. If that’s not a movie title it should be.
In the year 2017, NASA says we are on the verge of discovering extraterrestrial life. I say alien life forms are all around, and they might even help us clean up our environment. I call archaea aliens because some are extremeophiles and they may have been the original seeds that brought life to earth from comments.
Life on Earth is thought to have originated from the oldest single-cell archaea and bacteria.
Panspermia – The hypothesis that microorganisms may transmit life from outer space to habitable bodies; or the process of such transmission.
It’s just a theory, but there was a time red “cells” rained down on the earth, apparently from a meteor.
Now we have all these great ideas. Next, we  need the political will and organization to validate them, fund them, and then to get the projects rolling as massive multinational earth recovery efforts. I wish cleaning up the earth was a new worldwide sport.
Make it happen if you can.
Read More
[1] https://www.sciencedirect.com/science/article/abs/pii/S0045206824006795
[2] https://gizmodo.com/this-modified-microbe-could-turn-carbon-emissions-into-460737465
[3] https://www.pnas.org/doi/10.1073/pnas.1222607110
[4] https://www.sciencedirect.com/science/article/pii/S0958166921001233
[5] https://news.mongabay.com/2013/03/scientists-a-step-closer-toward-creating-biofuels-directly-from-atmospheric-co2/
[6] https://biomassmagazine.com/articles/research-may-result-in-biofuel-production-from-atmospheric-co2-8764
[7] https://www.uni-regensburg.de/biologie-vorklinische-medizin/mikrobiologie/news/index.html
[8] https://www.mpi-bremen.de/Jun.-Prof.-Dr.-mirko-basen-ancient-thermophiles-in-the-21st-century-a-new-era-for-heat-loving-microbes.html
[9] https://pmc.ncbi.nlm.nih.gov/articles/PMC4466265/
[10] https://wwwnc.cdc.gov/eid/article/30/8/24-0181_article
[11] https://asm.org/articles/2024/july/the-archaeome-emerging-player-health-disease
[12] https://pmc.ncbi.nlm.nih.gov/articles/PMC145348/
[13] https://www.nature.com/articles/s41598-017-04197-4
[14] https://pmc.ncbi.nlm.nih.gov/articles/PMC7043356/
[15] https://www.frontiersin.org/journals/microbiology/articles/10.3389/fmicb.2019.02796/full
[16] https://www.nature.com/articles/s41598-023-38710-9
[17] https://www.biorxiv.org/content/10.1101/334748v1.full-text